Cellular and molecular mechanisms underlying mitochondrial
structure and function in mammalian neurons
One of the most striking features of neurons is the extraordinary structural and functional compartmentalization of their somatodendritic and axonal domains. Neurons use this high degree of polarization to receive electrical and chemical information from many other neurons, integrate it, and then transmit this information to their synaptic partners in the form of action potentials. Recent work has started to define the molecular logic underlying neural development in vivo ranging from axon specification during neuronal polarization to terminal axon branching. At later stages, circuit activity is critical for proper development as it stabilizes newly formed branches. Results from our work and others has also revealed that the specific localization of mitochondria is required for both axonal and dendritic development and maintenance. The highly stratified patterns of axon and dendrite branching in vivo cannot be recapitulated in the widely used in vitro dissociated culture systems, and therefore we must begin to develop tools and methods to study these processes in vivo.
To begin establishing these in vivo tools I collaborated with Dr. Gergely Turi in Dr. Attila Losonczy’s lab (Columbia University) during my postdoc to set up a 2-photon imaging platform for visualizing mitochondrial dynamics in layer 2/3 cortical axons for the first-time in awake behaving mice. We discovered that by postnatal day 10 (P10), over 90% of mitochondria are already captured along the axon and that this ratio is maintained at P45 in vivo (published in Current Biology, 2016 (Video below)). These results argue that high levels of mitochondrial trafficking in axons occurs during development, but that in mature cortical neurons most mitochondria are anchored at specific points along axons, largely at presynaptic boutons. We believe this constitutes a ‘paradigm shift’ for the field, because so far it has focused almost exclusively on studying the molecular mechanisms underlying mitochondria trafficking along axons, while these results argue that it is equally important to understand how and where mitochondria are captured along the axon.
Video 1: Mitochondrial motility is low in layer 2/3 distal axons in vivo of P32 awake behaving mice (Top panel) Timelapse video of a tdTomato expressing layer 2/3 distal axon segment and its YFP-labeled mitochondria. Frames were acquired every 10 seconds for 10 minutes (Played at 10 fps). (Middle panel) The YFP channel from the axon segment above. (Bottom panel) Kymograph of the above video demonstrating the low motility of mitochondria in distal layer 2/3 cortical axons at P32.
Finally, mitochondrial morphology is vastly different in the somatodendritic domain (where they form an extended network) versus the axon (where they are short, individual units; ~1 micron in length). Based on this observation, we hypothesized that mitochondrial fission must be differentially regulated in the axon (fission dominant over fusion) relative to dendrites where mitochondrial fusion must be dominant over fission. We recently identified that mitochondrial fission factor (MFF) is the key regulator of mitochondrial fission in axons, and demonstrated that shRNA-mediated knockdown of MFF leads to a substantial increase in mitochondrial length in the axon, whereas its over-expression is sufficient to induce massive mitochondrial fission in dendrites. Increased mitochondrial length in the axon upon knockdown causes increased total calcium import into presynaptic mitochondria, decreased cytoplasmic calcium levels at presynaptic sites, reduced presynaptic vesicle release, and decreased branching in cortical axons (published in Nature Communications, 2018). This work, coupled with previous work from the Polleux and Kittler labs, demonstrates that mitochondrial size in axons is tightly regulated by MFF-dependent fission and that this high level of fission plays a critical role in regulating neurotransmitter release properties through mitochondria-dependent presynaptic calcium clearance.
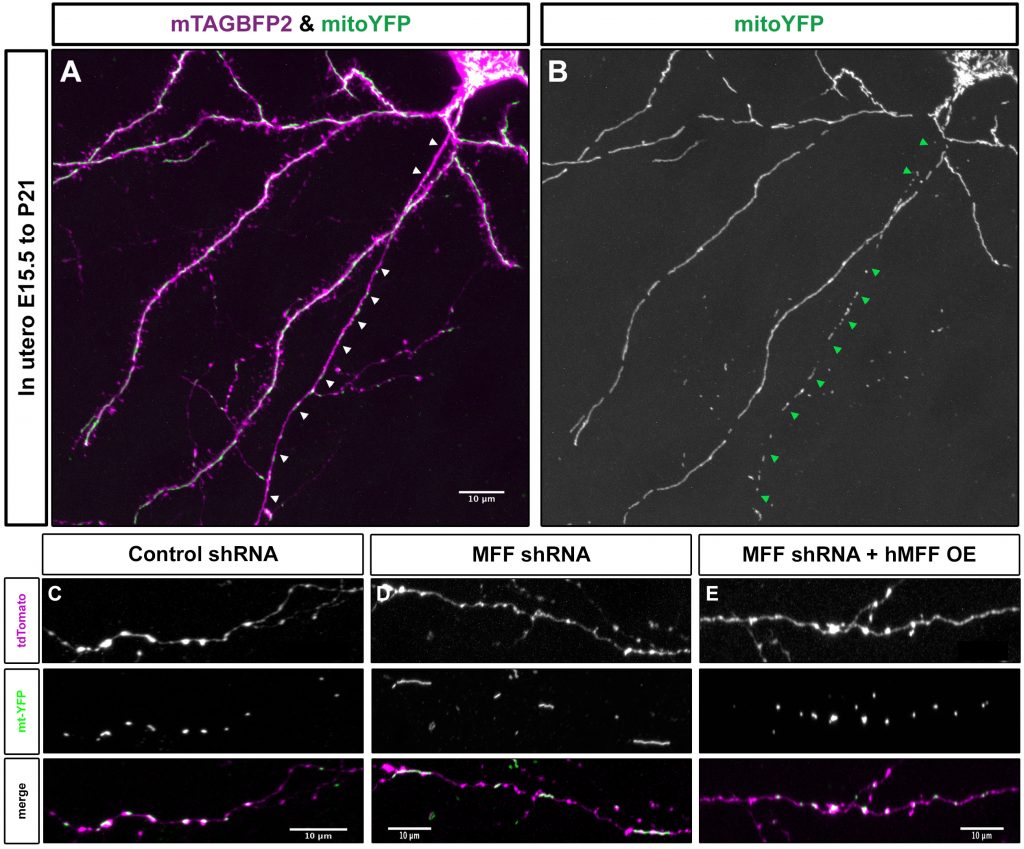
Figure 1: Mitochondria size in the axon is regulated via mitochondria fission factor (MFF). (A) Layer 2/3 cortical neuron labeled via in utero electroporation with mTAGBFP2 (to visualize the neuron) and mitochondrial targeted YFP (mitoYFP). (B) The YFP channel from the neuron in A. Arrowheads point to the axon. Mitochondria in the axon are small individual units, while dendritic mitochondria form elongated, tubular structures in vivo. (C) Mitochondria size in a control P21 layer 2/3 axon in vivo. (D) Mitochondria size in a MFF knockdown P21 layer 2/3 axon in vivo. (E) Mitochondria size in a MFF rescue P21 layer 2/3 axon in vivo. These results demonstrate that MFF activity is required for the small size of axonal mitochondria in vivo.
Project 1: Cellular and molecular mechanisms regulating neuronal subcellular mitochondrial morphology and function in vivo and their implications in neurodegeneration.
Mitochondrial fission and fusion play vital roles in maintaining the quality of mitochondria in neurons and have been implicated in many neurodegenerative diseases. Despite these reports, surprisingly little is known about the mechanisms that regulate the level of fission/fusion in neurons, and especially how mitochondrial fission/fusion can be differentially regulated in the axon and dendrites of the same neuron. Our experiments reveal that MFF is required to specify small mitochondrial size (<1 micron length) and function in layer 2/3 cortical axons in vitro and in vivo. Preliminary observations in CA1 pyramidal hippocampal neurons in vivo also establish that mitochondrial morphology can be regulated even within the dendritic compartment, as mitochondria are elongated and fused only in the distal apical tufts whereas short mitochondria are found in the rest of the dendritic tree (Figure 2). This observation is extremely interesting in light of the fact that these cell types receive different synaptic inputs onto the apical tufts versus the basal dendrites. Our working hypothesis based on these observations is that input-specific patterns of neuronal activity and/or compartment-specific signaling pathways control the level of MFF-dependent mitochondrial fission to tune the size of axonal and dendritic mitochondria, thereby allowing for compartment specific regulation of mitochondrial functions such as Ca2+ clearance and ATP production. We will use the tools that we have developed to interrogate this hypothesis, and to determine its role in models of neurodegeneration.
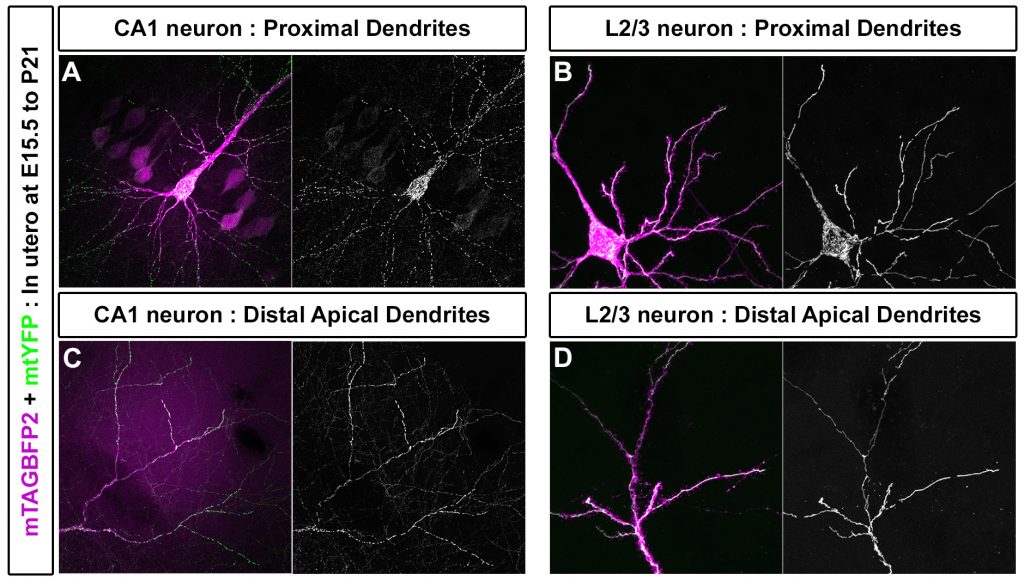
Figure 2: Mitochondria morphology is subcellularly regulated in the dendrites of CA1 hippocampal neurons in vivo. (A) Mitochondria in basal dendrites of CA1 hippocampal pyramidal neurons are small and fragmented. (B) In Layer 2/3 cortical neurons they are elongated and fused. (C) However, in the apical tufts of CA1 neurons mitochondria are elongated and fused much like in layer 2/3 cortical neurons (D). This demonstrates that compartmentalization of mitochondrial morphology is differentially regulated within the dendritic compartment of certain neuronal subtypes, and suggests that local neuronal activity or signaling pathways create distinct compartments of mitochondria structure and function.
Project 2: Identification and functional characterization of novel mitochondria-associated proteins in axons versus dendrites.
Recent work has shown that axonal mitochondria are associated with at least two unique proteins not found on dendritic mitochondria: Syntaphilin and TRAK1. These results imply that mitochondria found in axons and dendrites differ in terms of protein composition and function. To understand the mechanisms by which axonal mitochondria are compartmentalized in neurons, the field requires the development of novel methods that enable unbiased identification of proteins uniquely associated with axonal or dendritic mitochondria. To this end, we are developing and validating a method to differentially label axonal and somatodendritic mitochondria. We will use this approach to isolate and perform unbiased proteomics on axonal and somatodendritic mitochondria from pyramidal neurons in vitro and in vivo. Finally, we will develop new mouse lines based on this labeling which will give us the ability to tag axonal mitochondria in specific populations of neurons on a specific genetic background (i.e. mouse models of neurodegeneration). The identification of compartmentally unique mitochondria-associated proteins will allow us to characterize and understand the novel cell biological mechanisms differentially regulating mitochondria structure and function in the axonal vs. somatodendritc compartments. This new approach will also improve our understanding of novel mitochondrial targets involved in neurodegenerative diseases affecting the CNS and PNS